The design and planning of a multilayer PCB stackup are critical to achieving optimal performance, reliability, and manufacturability in complex electronic devices. A well-thought-out stackup reduces electromagnetic interference (EMI), enhances signal integrity, and ensures efficient power distribution. In this blog, we’ll explore the key considerations and best practices for planning a multilayer PCB stackup, offering insights that are valuable for PCB and PCBA professionals.
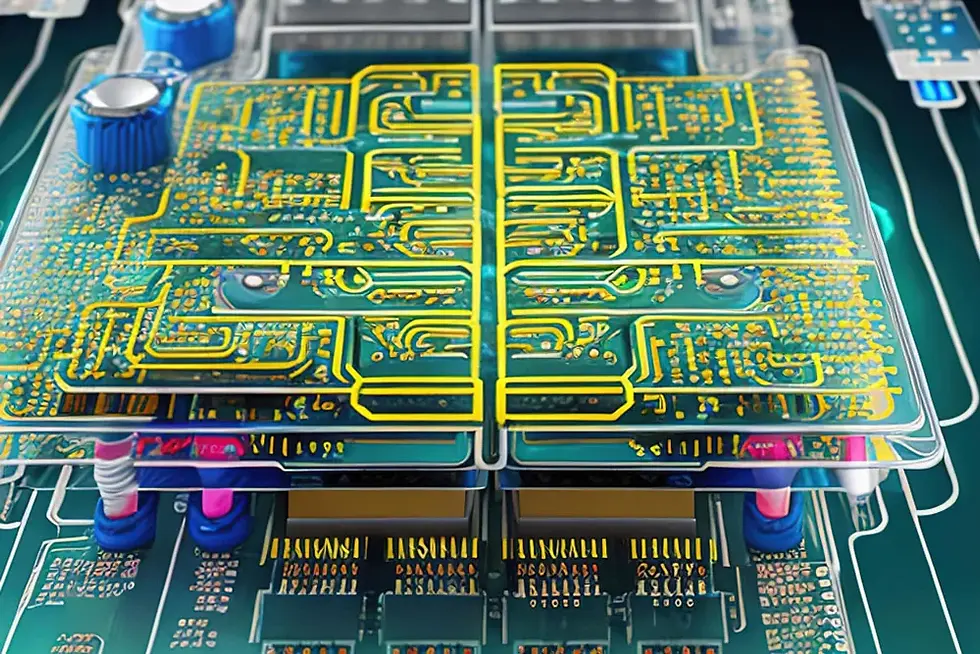
1. Understanding Multilayer PCB Stackup
A multilayer PCB stackup involves layering multiple conductive copper layers separated by insulating materials (dielectrics). These layers can include signal layers, power planes, and ground planes. The arrangement and configuration of these layers are crucial for controlling impedance, minimizing crosstalk, and ensuring overall circuit performance.
2. Key Considerations for Stackup Planning
Signal Integrity: Maintaining signal integrity is paramount in multilayer PCBs, especially in high-speed digital designs. Signal layers should be closely coupled with adjacent ground or power planes to minimize impedance variations and reduce noise.
Power Distribution: Power planes play a crucial role in distributing power efficiently across the PCB. Proper placement of power and ground planes helps in reducing voltage drops, minimizing power supply noise, and providing stable reference planes for signal layers.
Electromagnetic Compatibility (EMC): A well-planned stackup is essential for minimizing electromagnetic emissions and susceptibility. Placing signal layers between ground planes (known as stripline configuration) can significantly reduce EMI by containing electromagnetic fields within the PCB.
Thermal Management: Multilayer PCBs, especially those used in high-power applications, must consider thermal management in their stackup design. Heat dissipation can be enhanced by using thicker copper layers, incorporating thermal vias, and optimizing the placement of heat-generating components.
3. Common Stackup Configurations
4-Layer PCB Stackup: A typical 4-layer stackup consists of two signal layers and two planes (power and ground). The most common configuration is:
Top Layer: Signal 1
Layer 2: Ground Plane
Layer 3: Power Plane
Bottom Layer: Signal 2
This configuration is ideal for moderate-density designs where signal integrity and power distribution are important.
6-Layer PCB Stackup: A 6-layer stackup offers more flexibility and improved performance for high-speed designs. A common configuration is:
Top Layer: Signal 1
Layer 2: Ground Plane
Layer 3: Signal 2
Layer 4: Signal 3
Layer 5: Power Plane
Bottom Layer: Signal 4
This stackup allows for better signal routing, reduced crosstalk, and improved EMI control.
8-Layer PCB Stackup: For complex designs with high component density, an 8-layer stackup provides excellent signal integrity and EMI performance:
Top Layer: Signal 1
Layer 2: Ground Plane
Layer 3: Signal 2
Layer 4: Power Plane
Layer 5: Ground Plane
Layer 6: Signal 3
Layer 7: Power Plane
Bottom Layer: Signal 4
This configuration is commonly used in high-speed digital and RF designs where signal integrity and power distribution are critical.
4. Dielectric Material Selection
The choice of dielectric material impacts the overall performance of the PCB. Factors to consider include:
Dielectric Constant (Dk): A lower Dk material is preferred for high-frequency applications as it reduces signal delay and attenuation. Materials such as FR-4, Rogers, and polyimide are commonly used based on the application's frequency requirements.
Loss Tangent: The loss tangent (Df) of the dielectric material affects signal loss. For high-speed designs, materials with a low loss tangent are chosen to minimize signal degradation.
Thermal Properties: The thermal conductivity and glass transition temperature (Tg) of the dielectric material must be suitable for the operating environment and the thermal management needs of the PCB.
5. Impedance Control
Impedance control is vital for high-speed signal transmission. The stackup design must account for the impedance of signal traces, which is influenced by the trace width, spacing, dielectric thickness, and the proximity of ground planes. Tools like impedance calculators and field solvers are often used to design and verify the impedance of critical signal paths.
6. Manufacturability Considerations
While planning the stackup, it’s essential to consider the manufacturability of the PCB. Factors such as the number of layers, layer symmetry, and material selection affect the ease of fabrication and assembly. Working closely with your PCB manufacturer during the design phase ensures that the stackup is optimized for production, reducing costs and avoiding potential issues during manufacturing.
7. Best Practices for Stackup Design
Symmetry: Maintain symmetry in the stackup to avoid warping and bending during manufacturing. This is especially important in multilayer boards with an even number of layers.
Controlled Impedance: Ensure that impedance control is considered early in the design process to avoid costly revisions.
Power Integrity: Place power and ground planes as close as possible to reduce inductance and ensure stable power delivery.
Consultation with Manufacturer: Collaborate with your PCB manufacturer to ensure that the stackup design aligns with their fabrication capabilities and materials.
Conclusion
Planning a multilayer PCB stackup is a complex but essential aspect of PCB design that directly impacts the performance, reliability, and manufacturability of the final product. By carefully considering factors such as signal integrity, power distribution, EMC, thermal management, and manufacturability, you can create a stackup that meets the rigorous demands of modern electronic designs.
コメント